引用本文: | 朱崇抒,曹军骥,刘随心,屈 垚,张 婷.2016.陕南农村冬季PM2.5主要化学组分特征[J].地球环境学报,(5):494-500 |
| ZHU Chongshu, CAO Junji, LIU Suixin, QU Yao, ZHANG Ting.2016.The characteristics of chemical components for rural PM2.5 in winter over Shaannan[J].Journal of Earth Environment,(5):494-500 |
|
|
|
本文已被:浏览 5337次 下载 2828次 |
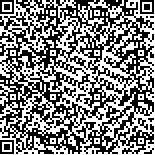 码上扫一扫! |
|
陕南农村冬季PM2.5主要化学组分特征 |
朱崇抒,曹军骥,刘随心,屈 垚,张 婷1,2
|
1.中国科学院地球环境研究所 中国科学院气溶胶化学与物理重点实验室,西安710061;2.中国科学院地球环境研究所 黄土与第四纪地质国家重点实验室,西安710061
|
|
摘要: |
通过对陕南农村冬季PM2.5采样分析,获得PM2.5质量浓度及主要化学组分特征。PM2.5平均质量浓度为89.5 ± 42.0 µg ∙ m−3,超过国家二级标准。观测期间PM2.5中OC、EC浓度平均值分别为16.0 ± 6.9 µg ∙ m−3和5.7 ± 3.2 µg ∙ m−3,OC/EC平均比值为3.0 ± 0.4。主要水溶性离子组分为NO3−, SO42−,和 NH4+。粒子数浓度与表面积浓度峰值主要集中在0.5 μm以下粒径段。PAHs、BeP和BaP平均质量浓度分别为48.9 ± 10.9 ng ∙ m−3、3.0 ± 0.9 ng ∙ m−3和1.2 ± 0.7 ng ∙ m−3,PAHs污染较严重,强致癌物BaP浓度超过国家环境空气质量标准年平均浓度限值。当地农村以石煤为主的能源结构及采用的燃烧方式是导致污染的重要因素。 |
关键词: PM2.5 化学组分 农村 陕南 |
DOI:10.7515/JEE201605006 |
CSTR:32259.14.JEE201605006 |
分类号: |
基金项目:国家自然科学基金项目(41271481) |
英文基金项目:National Natural Science Foundation of China (41271481) |
|
The characteristics of chemical components for rural PM2.5 in winter over Shaannan |
ZHU Chongshu, CAO Junji, LIU Suixin, QU Yao, ZHANG Ting1,2
|
1. Key Laboratory of Aerosol Chemistry & Physics, Institute of Earth Environment, Chinese Academy of Sciences, Xi’an 710061, China;2. State Key Laboratory of Loess and Quaternary Geology, Institute of Earth Environment, Chinese Academy of Sciences, Xi’an 710061, China
|
Abstract: |
Background, aim, and scope The usage of biomass and coal for cooking and heating is common in rural area in China. In reality, the emission largely contributes to the chemical components of particulates. The study here presented the levels of rural carbonaceous fractions, ions and PAHs over Shaannan. Materials and methods The observation campaign was conducted in a rural site at Shaannan. Samples were collected by using mini-volume samplers (Airmetrics, USA) operating with a flow rate of 5 L ∙ min−1 for 24 hours. All samples were collected on 47 mm Whatman quartz microfibre filters (QM/A). The filters were pre-heated before sampling at 800℃ for 3 hours. After collection, the filters were stored in a refrigerator before chemical analysis. Before and after field sampling, quartz filters were equilibrated for 24 hours in the box at a constant temperature (20℃ to 23℃) and relative humidity (35% to 45%). The PM2.5 mass was determined by weighing the filters before and after sampling on an electronic microbalance (1 μg sensitivity) (Sartorius, MC5, Germany). All the filters were analyzed for carbon fractions using a DRI Model 2001 Thermal/Optical Carbon Analyzer (Atmoslytic Inc., Calabasas, CA, USA). Carbon fractions were analyzed following the Interagency Monitoring of Protected Visual Environments (IMPROVE-A) thermal/optical reflectance (TOR) protocol. The method produced data for four OC fractions (OC1, OC2, OC3, and OC4 in a helium atmosphere at 140℃, 280℃, 480℃, and 580°C, respectively), a pyrolyzed carbon fraction (OP, determined when reflected laser light attained its original intensity after oxygen was added to the combustion atmosphere), and three EC fractions (EC1, EC2, and EC3 in a 2% oxygen/98% helium atmosphere at 580℃, 740℃, and 840℃, respectively). The IMPROVE protocol defined OC as OC1+OC2+OC3+OC4+OP and EC as EC1+EC2+EC3−OP. The analyzer was calibrated with known quantities of CH4 each day. Replicate analyses were performed once every ten samples. The blank filters were also analyzed for quality control and the sample results were corrected by the average of the blank concentrations, which were 0.96 µg ∙ m−3 and 0.23 µg ∙ m−3 for OC and EC, respectively. The concentrations of three anions (Cl−,NO3− and SO42−) and five cations (Na+, NH4+,K+, Mg2+ and Ca2+) were determined in aqueous extracts of the sample filters by using a Dionex-600 Ion Chromatograph (Dionex Inc., Sunnyvale, CA, USA). Standard solution and blank test were performed before sample analysis and the result of correlation coefficient of standard samples was more than 0.999. One in 10 extracts was reanalyzed and none of the differences between these replicates exceeded precision intervals. All the reported data of water solvable ions were corrected by the filter blanks. Minimum detection limits were as follows: 0.001 µg ∙ mL−1 for Na+, NH4+, K+, Mg2+ and Ca2+; 0.008 µg ∙ mL−1 for Cl−; 0.025 µg ∙ mL−1 for NO3−; and 0.027 µg ∙ mL−1 for SO42−. Traditional method for determining PAHs involve solvent extraction (SE) followed by gas chromatography/mass spectrometry (GC/MS). For our study, we used an in-injection port thermal desorption GC/MS method because it involves a short sample preparation time (<1 min), the procedure minimizes contamination from solvent impurities, and detection limits as low as a few nanograms of the target analytes can be achieved. Results The concentrations of rural PM2.5 were measured in winter over Shaannan. Levels of carbon species as well as OC/EC ratios are also obtained. The average concentrations of PM2.5, OC and EC were 89.5 ± 42.0 µg ∙ m-3, 16.0 ± 6.9 µg ∙ m−3, and 5.7 ± 3.2 µg ∙ m−3, respectively. The average OC/EC ratio of PM2.5 was 3.0 ± 0.4. The result shows a high correlation between OC and EC for the rural environment in winter (R = 0.98).The major water soluble inorganic ions were NO3−, SO42−, and NH4+. SO42−contributions were the highest of the ionic species of PM2.5, followed by NO3−. The concentrations of PAHs, BeP, and BaP were 48.9 ± 10.9 ng ∙ m−3, 3.0 ± 0.9 ng ∙ m−3, and 1.2 ± 0.7 ng ∙ m−3, respectively. Discussion The knowledge of the compositions of PM2.5 is critical for understanding and then for ameliorating the atmospheric environments. According to our observations, the site experienced heavy smoke from coal burning for cooking and heating, which were the major contributors to fine particulate emissions in winter. Considering the patterns of local energy consumption, effective control measures were proposed to reduce the emissions of local coal combustion for residential heating and cooking. Conclusions The discussion presented in this work could give implications for the future strategies and implementation of rural air quality improvement. Owing to the large population living in rural areas, the coal and agricultural fuel burning-activity in rural areas could significantly contribute to emissions inventories. Recommendations and perspectives Clean energy resources, such as wind and solar energy, are currently underutilized. Strategies and technology for improving energy efficiency and structure will be very important in reducing emissions in rural areas. |
Key words: PM2.5 chemical components rural area Shaannan |
|
|
|
|
|